“We continue to count on the valuable contributions of the Arms Control Association.”
A Win-Win Solution for Iran’s Arak Reactor
Ali Ahmad, Frank von Hippel, Alexander Glaser, and Zia Mian
In November 2013, Iran and the P5+1 group of countries (China, France, Germany, Russia, the United Kingdom, and the United States) agreed on a six-month Joint Plan of Action to enable negotiations on a final settlement to contain the proliferation risks from Iran’s nuclear program.
This interim agreement freezes Iran’s enrichment capacity, thereby preventing a further shortening of the time Iran would require to produce weapons quantities of highly enriched uranium (HEU) if it wished.1 This enrichment capacity has expanded greatly over the years since it first came to international attention in 2002.
Iran and the P5+1 also have agreed on the need to constrain Iran’s option to produce plutonium for weapons using the reactor that is under construction near the city of Arak and that will be under International Atomic Energy Agency (IAEA) safeguards.
Under the Joint Plan of Action, Iran has agreed to freeze the Arak reactor project for six months.2 It also has committed not to separate plutonium from spent nuclear fuel or construct a facility capable of doing so. These are important interim commitments.
According to Ali Akbar Salehi, the head of the Atomic Energy Organization of Iran, the Arak reactor is intended for radioisotope production and testing of nuclear fuel and materials. In response to the P5+1 proposal that Iran scrap the Arak reactor project, Salehi stated that “we see no point stopping the work on this reactor.” He has acknowledged, however, the international community’s concerns about the Arak reactor and offered the possibility of design changes “in order to produce less plutonium in this reactor and in this way allay the worries and mitigate the concerns.”3
This article proposes technical steps that would provide assurance that Iran could not quickly make sufficient plutonium for a nuclear weapon with the Arak reactor while at least maintaining the reactor’s performance in peaceful applications.
The solution proposed here involves changing the fueling and operating power of the Arak reactor to make it less of a proliferation concern. The case of Algeria’s Es-Salam research reactor provides a useful precedent.
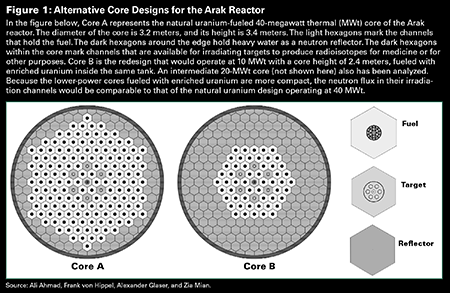
The U.S. discovery in 1991 that China was building a reactor in Algeria that, like the Arak reactor, would be fueled with natural uranium and use heavy water as a neutron moderator caused a diplomatic crisis. Based on satellite imagery, the United States estimated that the reactor’s power could be as high as 50 megawatts thermal (MWt), in which case, if it were fueled with natural uranium, it would be able to produce as much as “10 to 13 kilograms per year” of plutonium—more than enough for a simple nuclear weapon. China informed the United States, however, that the reactor’s design power would be 15 rather than 50 MWt and that the fuel provided would be enriched to 3 percent in the chain-reacting isotope uranium-235 rather than natural uranium, which contains only 0.7 percent U-235. The United States calculated that, with that fuel and power, the reactor would produce in its spent fuel “slightly less than 1 kilogram of plutonium per year.”4 With the additional commitment that the reactor would be placed under IAEA safeguards, the crisis was resolved. A similar solution is available for the Arak reactor.
The Arak Research Reactor
The 40-MWt Arak research reactor is a proliferation concern in part because other countries have used this type of reactor to produce plutonium for nuclear weapons (see box). Reactors that use natural uranium fuel are especially well suited for plutonium production because virtually all of the neutrons they produce that are excess to the requirements for maintaining the fission chain reaction are absorbed in the uranium-238, which constitutes 99.3 percent of the fuel. The addition of the neutron turns U-238 into uranium-239, which quickly decays into plutonium-239. Very few neutrons are left over for use in research and radioisotope production. To gain more neutrons, heavy-water reactors are designed to operate at high power, which requires more nuclear fuel and larger reactor cores.
Assuming that the Arak reactor operates at full power about 300 days per year, natural uranium fuel could be kept in its core for about 3.5 years. The analysis in this article assumes that during this period, the reactor would be refueled three times with one-third of the fuel in the reactor core replaced at each refueling. The discharged fuel would be placed in an on-site, water-filled cooling pool.
Under this scenario, the pool would contain 27 kilograms of unseparated plutonium after the third discharge. The fuel still in the reactor core could contain up to another 20 kilograms. Therefore, the plutonium inventory on the reactor site, including the amount in the reactor, would be up to 50 kilograms.5 Most of this plutonium would not be weapons grade, but it would be weapons usable.6 Using the IAEA estimate of 8 kilograms of plutonium for a first-generation nuclear weapon, 50 kilograms would be enough for about six weapons.
Before it could be used for a weapon, however, the plutonium in the spent fuel would have to be separated out in a remotely operated chemical reprocessing plant behind heavy shielding. Iran has repeatedly stated that it has no intention of building a reprocessing plant, and there is no evidence or even claim that it is doing so. Yet, there is the theoretical possibility that a small “quick and dirty” reprocessing plant could be constructed clandestinely. The IAEA assumes for the purpose of designing its safeguards that it would take one to three months to separate enough plutonium for a weapon.7
Furthermore, the Arak reactor is not the only potential source of plutonium in Iran. The Bushehr 3,000-MWt power reactor has much more plutonium in its core and in its spent fuel pool than Arak is likely to have. If an annual fuel reload were discharged early from the Bushehr reactor after the fuel achieved about 15 percent of its design burn-up (several months), it would contain about 50 kilograms of weapons-grade plutonium.8 The relatively large amount of plutonium in irradiated fuel at the Bushehr reactor is one reason why the IAEA is inspecting it quarterly.
The Light-Water Alternative
In 2005, because of their proliferation concerns about the Arak reactor, France, Germany, and the UK—the group that was the predecessor to the P5+1—offered to help Iran obtain a light-water research reactor instead.9 This is still the proposal of the P5+1 in its talks with Iran.
Such a reactor would most likely be a higher-power, more modern version of the Tehran Research Reactor, which the United States provided Iran in 1960 under the Atoms for Peace program. The Tehran facility is a 5-MWt, pool-type reactor whose fuel today is 19.75 percent enriched in U-235. This is just below the 20 percent threshold above which uranium is considered to be weapons usable. The core of the Tehran reactor is a small rectangular cuboid that sits at the bottom of a deep pool of “light” (ordinary) water. The water is pumped through the core to cool it and provides radiation shielding for workers using the reactor.
Box: Research Reactors and Nuclear Weapons Programs Reactors that are fueled with natural uranium and use heavy water as a neutron moderator have been used to produce plutonium for use in weapons since the Manhattan Project. Almost all of the neutrons that are produced beyond those required to sustain the uranium-235 fission chain reaction in the fuel are absorbed by uranium-238 to produce plutonium. U-238 is 140 times more abundant in natural uranium fuel than is U-235. The first heavy-water reactor, Canada’s 40-megawatt thermal (MWt) NRX, on which construction began in 1944, was originally designed to provide plutonium for the wartime U.S. nuclear weapons program.1 India, Israel, and Pakistan currently use reactors of this basic type to produce their weapons plutonium, and other countries have built such reactors for that purpose.
ENDNOTES 1. D.G. Hurst and A.G. Ward, “Canadian Research Reactors,” Atomic Energy of Canada, Ltd., 1956. 2. International Panel on Fissile Materials, “Global Fissile Material Report 2010,” December 2010, ch. 8, http://fissilematerials.org/library/gfmr10.pdf. 3. Ibid., ch. 9. 4. Ibid., ch. 10. |
Pool-type light-water research reactors are attractive because they are simple and their compact cores are intense sources of neutrons. Of the nine isotope production reactors worldwide in the power range of 10 to 30 MWt whose construction began in 1980 or later, eight are light-water pool-type reactors.10 The ninth is Algeria’s Es-Salam heavy-water reactor.
Light-water research reactors fueled with 19.75 percent-enriched uranium produce much less plutonium than reactors fueled with natural uranium because there are only about four U-238 nuclei in their fuel for every U-235 nucleus, a proportion much lower than the 140-to-1 ratio in natural uranium fuel. As a result, a 20-MWt light-water research reactor operated 300 days a year would produce only 0.7 kilograms of plutonium a year and would have on average about 1 kilogram of plutonium in its core.11 The IAEA must monitor the use of such reactors to ensure that natural uranium targets are not placed in or around the core to produce more plutonium.
At the same time, because such a small fraction of their neutrons is wasted on the production of plutonium, relatively low-power light-water research reactors can produce large quantities of medical radioisotopes. The operators of Australia’s multipurpose 20-MWt OPAL reactor, for example, plan to produce and mostly export 2,700 six-day curies per week of molybdenum-99, the primary radioisotope used in medicine.12 Iran currently imports from Russia 100 six-day curies of this product per week to supplement the production by the Tehran Research Reactor.13
Iran recognizes the efficiency of pool-type light-water reactors for research and radioisotope production. In February, Iran informed the IAEA that a “10 MW light water pool type research reactor with 20 percent enriched uranium oxide fuel, is planned to be constructed in order to fulfill the national demand on educational nuclear research, material testing, medical radio isotopes production and other beam line application.”14 It appears, however, that Iran intends to build this new reactor in addition to the Arak reactor rather than as an alternative.
The Arak reactor perhaps could be converted into a light-water pool-type reactor. If the pressure vessel were removed, it might be possible to flood the radiation shield chamber with water and install the core of a pool-type reactor at the bottom.
Iran takes pride in the Arak reactor, however, and is unwilling to scrap it in exchange for a light-water research reactor. Salehi emphasized its importance as a scientific and technological achievement.15 As he suggested, however, there exist plausible options for modest changes in the operation of the Arak reactor that would dramatically reduce its plutonium production without reducing its performance as a research reactor. These changes could be a basis for a mutually acceptable solution.
Reducing Plutonium Production
The amount of plutonium produced in the Arak reactor could be reduced drastically with the implementation of two complementary measures.
- Convert the reactor from using natural uranium fuel to low-enriched uranium fuel. For a given reactor power, the total quantity of U-235 in the fuel would not change because using less-dense fuel would compensate for the increased enrichment. The quantity of U-238, however, would be greatly reduced, and the quantity of plutonium produced would be reduced almost in proportion.16
- With low-enriched fuel, the power could be reduced to 20 or even 10 MWt, further reducing plutonium production. With a proportionately smaller core, the reactor still would have at least as large a capacity to produce neutrons for medical isotopes and for scientific research as the current design, a 40-MWt reactor fueled by natural uranium.
These options were examined for variations of Arak’s current core design (fig. 1 [Core A]). Because Iran has not publicly released the full design details of the Arak reactor, the 40-MWt design created by a group of Norwegian researchers, based on descriptions in Iranian publications, is used for reference here.17 The core contains 8.7 metric tons of natural uranium in the form of 10 metric tons of uranium dioxide.18
The analysis below compares the performance of this design with that of two possible alternative smaller cores in the same reactor vessel with heavy water as a moderator but with the power level reduced to 20 or 10 MWt and the fuel at each power level enriched to 5 or 19.75 percent U-235.
Converting the Arak reactor to 5 percent-enriched fuel might be preferable to converting to 19.75 percent-enriched fuel. If Iran produces its own fuel for the Arak reactor, it would be better that it not have a reason, in the near term at least, to produce more uranium that is enriched to almost 20 percent. Uranium enriched to that level requires much less additional enrichment to reach weapons grade (an enrichment level of 90 percent or more). Iran has produced enough uranium enriched to almost 20 percent to fuel the Tehran Research Reactor for several years at least and has suspended further production as a confidence-building measure under the Joint Plan of Action.
The need to produce 5 percent-enriched uranium for Arak could not be used by Iran to legitimize a large enrichment capacity. About 1,300 of Iran’s first-generation centrifuges, the IR-1, could produce enough material to fuel the Arak reactor operating at 20 MWt.19 It would take twice as much enrichment capacity to provide enough 19.75 percent-enriched uranium to fuel a light-water research reactor with the same power.
With regard to plutonium production in the fuel of the various cores, the analysis conducted for this article found that when fueled with 5 percent-enriched uranium, the Arak reactor, operating at 20 MWt, would produce no more plutonium in its fuel than a light-water research reactor of the same power fueled with 19.75 percent-enriched uranium (table 1). If the Arak reactor were fueled with 19.75 percent-enriched uranium, the amount of plutonium produced in its fuel would be one-quarter the amount produced in the core of a light-water research reactor with the same power.
It is well understood how to change the enrichment of a research reactor’s fuel without changing the geometry of the fuel or core. Between 2001 and 2010, 20 research reactors were converted from using weapons-grade uranium fuel to 19.75 percent-enriched fuel.20 That was a reduction of fuel enrichment rather than the increase for Arak discussed here, but the approach is the same. The analysis for this article has examined the effect of reducing the concentration of uranium in the fuel while the enrichment is increased so that the amount of U-235 in the fuel stays constant. This would require Iran to make a different kind of fuel for Arak than the uranium dioxide fuel that is currently planned.
Fuels currently used in most research reactors are made by dispersing uranium-containing material in aluminum to make the “meat” of the fuel and then sealing that meat inside aluminum cladding to isolate the uranium and its fission products from the cooling water. Iran already is producing one such fuel for the Tehran Research Reactor in which the uranium is in the form of the uranium oxide U3O8.21 This fuel can be produced with uranium densities as high as 3.2 grams of uranium per cubic centimeter.22 Five percent-enriched fuel for the Arak reactor would require a uranium density of about 1.3 grams per cubic centimeter, which would be relatively straightforward to fabricate.
Converting to enriched uranium fuel would make more neutrons available for radioisotope production and other purposes. Arak reportedly is designed with eight channels reserved for purposes such as irradiating radioisotope production targets and test fuel.23 A much smaller 5 percent-enriched core could produce as many neutrons for such purposes operating at 10 MWt as could the large natural uranium core operating at 40 MWt.24
In summary, any of the redesigns of the Arak reactor suggested here would reduce plutonium production to less than 1 kilogram per year, comparable to the reduction that would be accomplished by replacing the Arak reactor with a light-water research reactor. At the same time, these redesigns would not reduce the usefulness of the reactor for making radioisotopes and conducting research. Thus, this approach would meet Iran’s needs and would address the concerns of the international community as reflected by the P5+1.
To prevent the accumulation of large amounts of plutonium-bearing spent fuel at Arak, the spent fuel could be shipped out after a few years. Russia already has agreed to remove the spent fuel from Iran’s Bushehr reactor after it has cooled sufficiently. Ordinarily, spent fuel from light-water power reactors is not shipped until it has cooled for five years, but it can be shipped sooner with a reduced loading of fuel per transport cask. The Bushehr reactor will discharge about 27 metric tons of spent fuel a year. It would be a minor matter to add to the shipments of its spent fuel the approximately 0.1 to 0.2 metric tons of 5 percent-enriched spent fuel that would be discharged annually by the Arak reactor operating at 10 to 20 MWt.
Breakout Potential
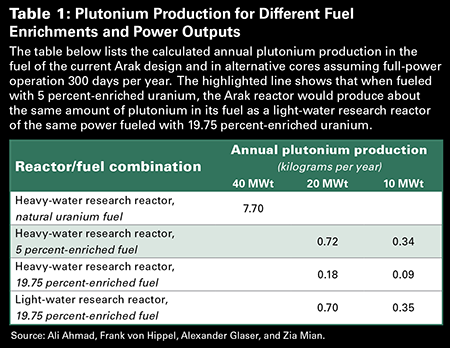
The overarching goal of the P5+1 negotiations with Iran is to limit Iran’s potential to quickly produce enough plutonium or HEU for a weapon. A relevant question is how the breakout potential of an Arak reactor fueled with enriched uranium and operating at reduced power would compare with the proliferation risk from Iran’s uranium-enrichment program.
The small amount of plutonium in the 5 percent-enriched spent fuel means that, to make a weapon quantity of plutonium in a hurry, Iran first would have to manufacture about 2,700 natural uranium fuel rods for the reactor. It then would replace its enriched fuel with this natural uranium fuel and operate the reactor at 40 MWt continuously for nine months. These actions would be quickly detected by IAEA inspectors. The fabrication of the natural uranium fuel would provide additional warning unless Iran had established a clandestine fuel-fabrication plant. After that, it would take months to separate the plutonium even if Iran had clandestinely constructed a reprocessing plant. The total breakout time would be at least a year.
For comparison, the Institute for Science and International Security estimates it would take Iran’s enrichment program significantly less than six months to produce one weapon’s worth of HEU.25 To increase this breakout time to six months, Iran would have to reduce its enrichment capacity from the equivalent of the approximately 19,000 IR-1 centrifuges it had operating or installed in early February to the equivalent of only 4,000 IR-1 centrifuges.26
Thus, if the Arak reactor is converted to operate on enriched uranium at reduced power, it is likely to be less of a breakout threat than Iran’s enrichment program.
The conversion steps described above are technically feasible and would not reduce Arak’s usefulness for civilian purposes. They provide a sound basis for resolving one of the key points of contention in the talks on Iran’s nuclear program.
Ali Ahmad, Frank von Hippel, Alexander Glaser, and Zia Mian are members of the Program on Science and Global Security at Princeton University.
ENDNOTES
1. See International Atomic Energy Agency (IAEA), “Communication Dated 27 November 2013 Received From the EU Representative Concerning the Text of the Joint Plan of Action,” INFCIRC/855, November 27, 2013 (contains the Joint Plan of Action as an attachment) (hereinafter Joint Plan of Action); Office of the Press Secretary, The White House, “Summary of Technical Understandings Related to the Implementation of the Joint Plan of Action on the Islamic Republic of Iran’s Nuclear Program,” January 16, 2014, http://www.whitehouse.gov/the-press-office/2014/01/16/summary-technical-understandings-related-implementation-joint-plan-actio.
2. Joint Plan of Action, fn. 3.
3. “Arak Heavy Water Reactor Is for Peaceful Research: Dr. Salehi (Part 2),” Press TV, February 5, 2014, www.presstv.ir/detail/2014/02/05/349340/false-allegations-wont-stop-arak-reactor/.
4. U.S. Department of State, “Talking Points on Algerian Nuclear Developments for Delivery to Senators Glenn and Roth (SGA), Senators Pell and Helms (SFRC), and Congressmen Fascell and Broomfield (HFAC),” July 29, 1991, http://www2.gwu.edu/~nsarchiv/nukevault/ebb228/Algeria-18.pdf.
5. It would be 47 kilograms with a full core in the pool and just before discharge of an additional one-third of a core.
6. The plutonium isotope produced by neutron capture in U-238 is plutonium-239. If this isotope absorbs a second neutron, there is about a 27 percent chance that it will not fission but rather turn into plutonium-240. In the United States, weapons-grade plutonium is defined as containing less than 7 percent plutonium-240. See U.S. Department of Energy, “Plutonium: The First 50 Years,” DOE/DP-0137, February 1996, p. 17. The fuel discharged from the Arak reactor after 3.5 years would contain 21 percent plutonium-240. With regard to the weapons usability of non-weapons-grade plutonium, the Department of Energy has written,
At the lowest level of sophistication, a potential proliferating state or subnational group using designs and technology no more sophisticated than those used in first-generation nuclear weapons could build a nuclear weapon from reactor grade plutonium [containing at least 19 percent plutonium-240] that would have an assured reliable yield of one or a few kilotons (and a probable yield significantly higher than that). At the other end of the spectrum, advanced nuclear weapon states such as the United States and Russia, using modern designs, could produce weapons from reactor-grade plutonium having reliable explosive yields, weight and other characteristics generally comparable to those of weapons made with weapon-grade plutonium.
U.S. Department of Energy, “Nonproliferation and Arms Control Assessment of Weapons-Usable Fissile Material Storage and Excess Plutonium Disposition Alternatives,” DOE/NN-0007, January 1997, pp. 38-39.
7. IAEA, “IAEA Safeguards Glossary, 2001 Edition,” International Nuclear Verification Series, No. 3 (2002), p. 22, table 1.
8. These calculations assume 2 grams per kilogram of uranium in 27 metric tons of fuel with an average burn-up of 5 MWt-days per kilogram.
9. IAEA, “Communication Dated 8 August 2005 Received From the Resident Representatives of France, Germany and the United Kingdom to the Agency,” INFCIRC/651, August 8, 2005.
10. The eight pool-type light-water reactors are OPAL (Australia, 20 MWt, 2002), FRM-II (Germany, 20 MWt, 1996), ETRR-2 (Egypt, 22 MWt, 1992), HANARO (South Korea, 30 MWt, 1987), JRR-3M (Japan, 20 MWt, 1985), RSG-GAS (Indonesia, 30 MWt, 1983), IRT-1 (Libya, 10 MWt, 1980), and RP-10 (Peru, 10 MWt, 1980). IAEA, “Research Reactors,” http://nucleus.iaea.org/RRDB/RR/ReactorSearch.aspx?rf=1.
11. Alexander Glaser, “On the Proliferation Potential of Uranium Fuel for Research Reactors at Various Enrichment Levels,” Science and Global Security, Vol. 14, No. 1 (2006): 10, table 4 (assuming 40 percent burn-up of the U-235 in the fuel).
12. National Research Council, “Medical Isotope Production Without Highly Enriched Uranium,” January 14, 2009, p. 44; “Australia Greenlights ‘Mega Moly’ Project,” Nuclear Engineering International, January 1, 2013, www.neimagazine.com/features/featurebox-australia-greenlights-mega-moly-project/. Six-day curies measure the quantity of molybdenum-99 left a week after it has been produced in a reactor. Assuming 10 percent losses and one day of decay during extraction from the neutron “target,” 15 percent of the molybdenum-99 in the target would remain after an additional six days of decay during shipping and use at a hospital.
13. “Russia Ready to Supply Iran With Medical Isotopes Molybdenum and Iodine,” Interfax Azerbaijan, February 22, 2011, http://interfax.az/view/458081 (in Russian).
14. IAEA, “Implementation of the NPT Safeguards Agreement and Relevant Provisions of Security Council Resolutions in the Islamic Republic of Iran,” GOV/2014/10, February 20, 2014.
15. Ali Akbar Salehi, head of the Atomic Energy Organization of Iran, in response to the question, “Why do you want this thing, why do you want this whole international, Western dispute over this particular issue [the Arak reactor]?” said, “First of all it is a scientific achievement, it is a technological achievement.” “Arak Heavy Water Reactor Is for Peaceful Research.”
16. The reduction is not exactly in proportion because, with a lower density of U-238, the nuclei shield one another from neutrons to a lesser degree.
17. Thomas Mo Willig, Cecilia Futsaether, and Halvor Kippe, “Converting the Iranian Heavy Water Reactor IR-40 to a More Proliferation-Resistant Reactor,” Science & Global Security, Vol. 20, Nos. 2-3 (October 2012): 97-116. For more detail, see Thomas Mo Willig, “Feasibility and Benefits of Converting the Iranian Heavy Water Reactor IR-40 to a More Proliferation-Resistant Reactor,” Norwegian University of Life Sciences, 2011. This group also examined the possibility of converting the Arak reactor to low-enriched uranium but limited itself to uranium dioxide fuel.
18. Willig, “Feasibility and Benefits of Converting the Iranian Heavy Water Reactor IR-40 to a More Proliferation-Resistant Reactor,” p. 66.
19. This assumes that the capacity of the first-generation centrifuges is 0.9 separative work units per year, the depleted uranium produced has an assay of 0.4 percent, the core contains 600 kilograms of 5 percent-enriched uranium, and the fuel life in the reactor is three years.
20. Ferenc Dalnoki-Veress, “Primarily Positive Perceptions: A Survey of Research Reactor Operators on the Benefits and Pitfalls of Converting From HEU to LEU” (paper presented at the European Research Reactor Conference, Ljubljana, Slovenia, April 1, 2014).
21. IAEA, “Implementation of the NPT Safeguards Agreement and Relevant Provisions of Security Council Resolutions in the Islamic Republic of Iran,” para. 58 and annex 2, tables 5 and 6.
22. Argonne National Laboratory, “Currently-Qualified Fuels,” July 29, 2008, http://www.rertr.anl.gov/QualFuel.html.
23. Mehdi Hashemi-Tilehnoee, Ali Pazirandeh, and Saman Tashakor, “HAZOP-Study on Heavy Water Research Reactor Primary Cooling System,” Annals of Nuclear Energy, Vol. 37, No. 3 (March 2010): 428-433.
24. The cumulative yield of 2.74-day half-life molybdenum-99 from thermal fission of U-235 is 6.11 percent. T. R. England and B. F. Rider, “Evaluation and Compilation of Fission Product Yields, 1993,” ENDF-349, LA-UR-94-3106, October 1994. For 20 percent-enriched uranium metal targets 0.5 millimeters thick irradiated for seven days, a one meter-high stack of targets containing 116 grams of uranium in the central channel would produce 1,800 six-day curies a week.
25. Assuming a large stock of 3.5 percent-enriched uranium and 68 kilograms of 19.75 percent uranium (equivalent to 100 kilograms of uranium hexafluoride) as feedstock. Institute for Science and International Security, “Defining Iranian Nuclear Programs in a Comprehensive Solution Under the Joint Plan of Action,” January 15, 2014, http://www.isisnucleariran.org/assets/pdf/Elements_of_a_Comprehensive_Solution_20Jan2014_1.pdf.
26. For the numbers of Iran’s installed centrifuges as of February 2014, see IAEA, “Implementation of the NPT Safeguards Agreement and Relevant Provisions of Security Council Resolutions in the Islamic Republic of Iran.” To get the number of first-generation equivalents, it has been assumed that each second-generation IR-2M centrifuge is the equivalent of five first-generation IR-1 centrifuges.